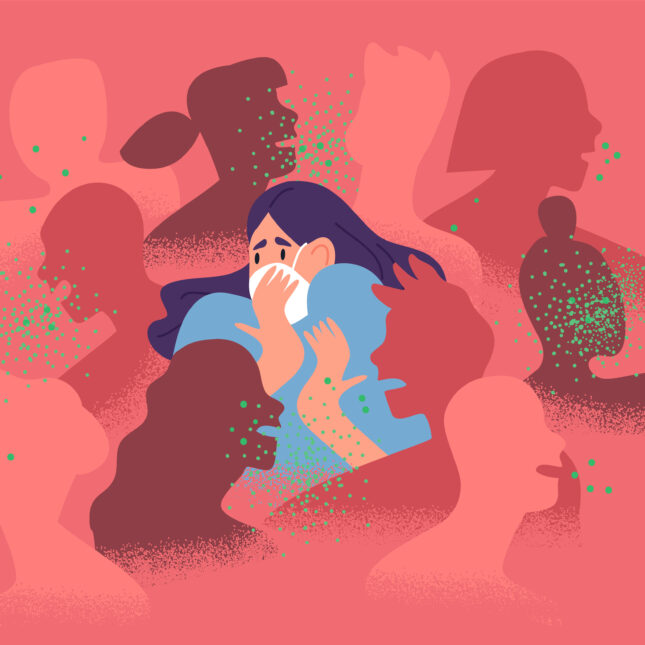
Carbon dioxide monitors have been around for decades. But in 2020, they became, almost overnight, a hot commodity. All of a sudden, people wanted them to help assess the safety of indoor spaces — to gauge the likelihood of breathing in coronavirus-laced particles that until very recently had been in someone else’s lungs.
No sensor can monitor how many infectious aerosols are swirling around us in real time. But carbon dioxide, or CO2, can act as a convenient proxy. People exhale it when they breathe, and in spaces that aren’t well ventilated, the gas accumulates. High CO2 concentrations can provide a warning sign that a lot of the air you’re inhaling is coming out of other people’s respiratory tracts.
For decades, that’s how aerosol scientists and ventilation engineers have mostly thought about CO2 — as a sort of indicator for the health of indoor environments. But over the last three years, researchers in the U.K. working with next-generation bioaerosol technologies have discovered that CO2 is more than a useful bystander. In fact, it plays a critical role in determining how long viruses can stay alive in the air: The more CO2 there is, the more virus-friendly the air becomes.
It’s a revelation that is already transforming the way scientists study airborne pathogens. But on a planet where burning fossil fuels and other industrial activities inject 37 billion metric tons of CO2 into the atmosphere each year, it could also have huge implications for human health.
“By increasing the CO2 in the air, we’re getting rid of a natural means by which viruses become inactivated,” said Allen Haddrell, an environmental chemist at the University of Bristol Aerosol Research Center, who led the new work. “It’s fascinating, but it’s also horrifying.”
Haddrell has spent his career designing instruments to better understand the complex chemical reactions that take place in the violent moments after someone coughs, sneezes, or breathes respiratory particles out. The reactions within those flecks determine how far they can float and how long they can linger in the air. In 2013, Haddrell was approached by the U.K.’s Defense Science and Technology Laboratory about making a machine to study how long bacteria can live inside these particles.
For the better part of a century, scientists have done those experiments with an instrument called a nebulizer, which creates a cloud of aerosols from liquids and releases them into a rotating drum. Then they wait and take time-stamped samples of the air inside the drum to calculate a given pathogen’s half-life: how long it can stay alive and aloft.
The trouble is, these nebulizers create complex plumes of particles, with different sizes and ages, so it can be difficult to know exactly what’s being measured. All the tumbling can give microbes a beating, too, making it difficult to interpret data about their viability. The British government’s military research arm wanted better information to help it prepare for a potential bioterrorism attack.
Making an instrument that could provide such information would turn into a six-year slog. Initially, Haddrell tried to adapt a technology known as “optical tweezers,” developed by a colleague of his at Bristol named Jonathan Reid. It uses lasers to trap particles in a microscope for extended periods of time, allowing researchers to measure the light scattering off of them to determine their physical and chemical properties. But bacteria — with all their proteins, fats, and other biomolecules — created patterns too complicated to fully understand.
Eventually, Haddrell, Reid, and a small team of Ph.D. students and postdocs turned to piezoelectronics. They could harness tiny charges of electricity to force fluid out under carefully controlled pressure, forming a perfect liquid bead — each with exactly the same chemical composition, size, and number of viral particles.
Instead of a rotating drum, Haddrell’s team built an electrodynamic force field to trap each tiny particle in mid-air at the moment of its creation. Using a laser and a camera, they could watch each hovering particle move and change as they exposed it to different environmental conditions. Once they’d measured the particles’ size and chemical composition, they could turn off the electrodynamic field, sending the beads plummeting into a Petri dish to be cultured.
The researchers called the contraption CELEBS, for “controlled electrodynamic levitation and extraction of bioaerosols onto a substrate,” and by 2019, they’d shown it could work quite well for benchmark bacteria like E. coli. The plan was to move on to other, more concerning airborne bacterial threats.
Then, a novel coronavirus emerged in China and began sickening millions of people all over the world.
On March 23, 2020, the same day Prime Minister Boris Johnson announced a nationwide lockdown to curb the widening outbreak of SARS-CoV-2, Haddrell got an email from a colleague at Bristol’s veterinary school, located on a farm about a 40-minute drive south. They were standing up a Containment Level 3 laboratory there and assembling a multidisciplinary rapid response team to study the virus. Could he get his CELEBS system working there, so they could learn more about how it behaved in respiratory particles?
“It was wild,” recalled Haddrell. “I went from thinking I would be home for the next three to six months to driving down there the next day.”
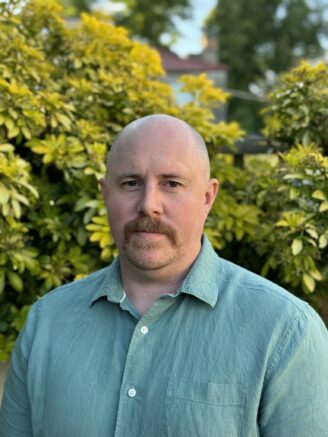
As the country shut down and the roads emptied of cars, Haddrell began building a high-containment version of his machine. While he did that, a postdoc named Henry Oswin took an existing CELEBS and got it calibrated to work with a mouse virus so they’d be ready to move on to SARS-CoV-2 as soon as the lab opened.
Around that time, researchers using traditional rotating drums began to report the first survivability data for the new coronavirus. One group, at the National Institute of Allergy and Infectious Diseases, found SARS-CoV-2 could stay viable in aerosols for up to three hours. Another, from Tulane University, found it could live in the air for over 16 hours. The divergent findings added to the confusion as officials crafted public health policies in those first months of the pandemic — when Americans were encouraged first not to wear medical-grade masks, then to wear homemade cloth coverings.
But when Haddrell’s team finally began their own experiments with SARS-CoV-2 in the fall of that year, they found the virus lost 90% of its ability to infect people within 20 minutes — with most of the loss occurring within the first five minutes.
Haddrell knew that going public with this information was going to be tricky. Because it had come from a totally novel technology, it would be easy to disregard the data as an artifact of an untested method. He wanted to make sure they had an explanation for why, in their hands, the coronavirus decayed much more quickly. So his team spent another year doing experiments and arguing over hypotheses on the daily drives to and from the new lab. Finally, they settled on a possible answer.
In biology labs, viruses are kept alive inside of cell cultures, where the byproducts of eating and growing can drive up the pH to dangerously alkaline levels. To keep that from happening, most culture media contains dissolved bicarbonate, which together with the carbon dioxide gas inside an incubator creates a buffering system.
Inside a nebulizer, though, these virus-laced bicarbonate solutions become aerosolized, releasing CO2 that builds up if it’s in a confined space. Haddrell’s team found that a standard nebulizer spraying cell culture media could raise the concentration of CO2 in a 7-liter box from 700 parts per million to over 1,100 over the course of 10 minutes. That extra CO2 acted like a buffer keeping the particles closer to a neutral pH, where respiratory viruses are quite content.
In the CELEBS system, that didn’t happen. As particles lost water through evaporation, they rapidly became more basic, which killed any SARS-CoV-2 viruses inside. Haddrell and his team proposed that pH, more than temperature and humidity, was the driving force behind viral decay in respiratory particles. And the method most widely used to study that had been incidentally altering pH without anyone realizing it.
If they were right, it would call into question decades of data produced by virology research groups around the world. So when Haddrell’s team posted their own results as a preprint in January 2022, they expected a bit of blowback. What they got was a hurricane.
“The headline is VERY convenient for people in power” tweeted Jose-Luis Jimenez, an atmospheric chemist at the University of Colorado, referencing a Guardian article about the research. “Now they can quote an aerosol preprint to keep doing nothing… [Wonder if they’ll forget not reviewed?]” Other leading aerosol scientists quickly piled on.
Haddrell, who wasn’t active on the social media site at the time, had unknowingly waded into a blistering online feud among researchers about the extent to which SARS-CoV-2 was airborne. If it could travel through the air and infect people via inhalation, even over short distances, then social distancing and handwashing wasn’t going to be enough to curb its spread — masking and ventilation would also be required.
The controversy hinged on differences in how aerosol scientists and medical professionals used words like “aerosol” and “airborne,” differences the World Health Organization only recently resolved. But in 2022, many feared that at the precise time when the Delta variant was filling up hospital wards across the U.K. with Covid patients, Haddrell’s data would be wrongly interpreted as casting doubt on the airborne route of transmission.
“That’s not what we were saying at all,” Haddrell said. “When Fox News emailed us, we knew we had a problem,” he said.
So when his group used CELEBS to systematically tease apart all the fundamental processes that could be causing SARS-CoV-2 to die in respiratory particles — temperature, humidity, salt content — and still found that pH played the biggest role, they didn’t put out the results as a preprint before it was published last summer. He also took to X, formerly Twitter, to explain what this finding meant in practical, pandemic terms: “Anything that reduces the acid content of the air will lower the aerosolized viral load #ventilation,” he wrote.
That’s because the human body is not unlike those nebulizers. We, too, carry around dissolved CO2 in the form of bicarbonate, which our blood transports from all our remote tissues into our lungs. When we exhale, and the bicarbonate inside our respiratory particles comes into contact with the outside air, it quickly breaks down and escapes as CO2 gas. If we’re in an enclosed space, that CO2 sticks around, limiting how high the pH inside those particles can climb — just like inside the rotating drums.
But that still left the question: How much CO2 is necessary to boost the odds of viral survival? The latest paper from Haddrell’s group, published in Nature Communications in April, found a disconcerting answer: not nearly as much as you might think. Increasing the CO2 concentration to just 800 parts per million, a level widely identified as being a marker of good ventilation, significantly extended the life spans of multiple SARS-CoV-2 variants. At higher concentrations — like those you might expect to find in a crowded, poorly ventilated room — Haddrell’s team found that 10 times as much virus remained infectious after 40 minutes when compared to clean air.
Together, the papers make a case for the need to improve the quality of indoor air, said Josh Santarpia, an expert in aerosol transmission of disease at the University of Nebraska Medical Center who was not involved in the study. “It’s not just that your exposure is higher, but the risk is also higher because there are more things that are staying alive,” he said. “It demonstrates the compounded nature of poor ventilation.”
Jimenez, who had been critical of Haddrell’s 2022 preprint and the subsequent media coverage, described the Bristol group’s latest work as “very important” in the push for stronger policies that regulate indoor air. In March, he and a group of international experts writing in Science presented a blueprint for national indoor air standards in public buildings, aiming to reduce both disease transmission and indoor pollutants.
In its call to action, the group proposed CO2 as one of three key metrics, suggesting ventilation rates that would keep CO2 levels at 800 parts per million or below. Lidia Morawska, an aerosol expert at Queensland University of Technology who was also an author on the Science perspective, told STAT that the Bristol work indicates that number might be too conservative.
“This is another argument for lowering the concentration of CO2 as much as we can,” Morawska said. “It’s moving from a role of being just a proxy to being understood as an enhancer, if we can use this term, for infection.”
Other aerosol experts who have studied SARS-CoV-2 extensively, like Linsey Marr at Virginia Tech, aren’t convinced that CO2 and pH are the whole story. “There are still many details we don’t understand about virus survival in respiratory particles,” she told STAT via email. “Their explanation might be right, but it might not.”
Haddrell agrees that more work needs to be done to understand how widely generalizable the phenomenon is. His team is now gearing up to begin more experiments with influenza A viruses.
But others are also taking up the reins. The Bristol group has now sent CELEBS machines to a handful of other labs, including Morawska’s in Australia and some in the U.S. With the recent spread of H5N1 bird flu into many mammal species, including dairy cattle and farmworkers who care for them, and the continuing rise in atmospheric CO2 levels, understanding the complex interplay between viruses, human bodies, and the environments where they eat, sleep, and breathe, is only growing more urgent.
To submit a correction request, please visit our Contact Us page.
STAT encourages you to share your voice. We welcome your commentary, criticism, and expertise on our subscriber-only platform, STAT+ Connect